Table of Contents
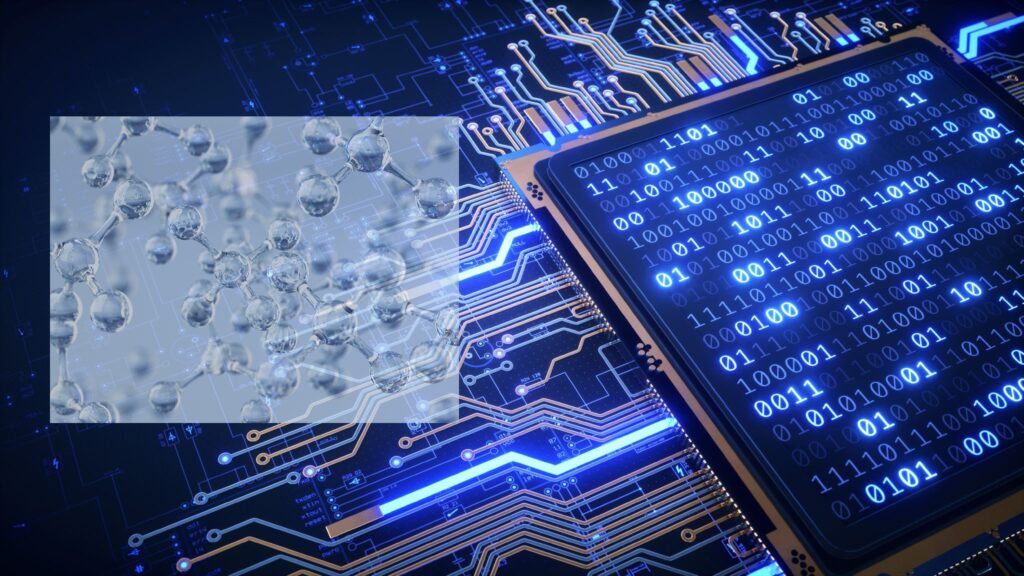
Introduction to Multi-omics
The scientific community is in the midst of a revolution, with integrative multi-omics standing at the forefront of transformative research. By merging various “omics” disciplines, researchers can unravel the complexities of biological systems with unprecedented precision. This article explores how integrative multi-omics is breaking barriers, advancing our understanding of biology, and paving the way for groundbreaking discoveries in health and disease.
In recent years, the field of omics has expanded significantly, encompassing genomics, proteomics, transcriptomics, metabolomics, and more. Each “omics” layer provides a unique perspective on the molecular underpinnings of life. Genomics, for instance, focuses on the DNA sequence of organisms, while proteomics examines the protein landscape.
Despite the value of individual omics studies, they offer only a fragmented view of biological systems. Integrative multi-omics aims to synthesize data from various omics layers, providing a holistic understanding of biological processes and disease mechanisms. This comprehensive approach enables researchers to uncover interactions and regulatory mechanisms that would otherwise remain hidden.
The Different Omics Layers
To appreciate the impact of integrative multi-omics, it’s essential to understand the distinct layers it encompasses.
Genomics
Genomics involves the study of an organism’s complete set of DNA, including all of its genes. Techniques like whole-genome sequencing (WGS) and genome-wide association studies (GWAS) have revolutionized our understanding of genetic variations and their role in health and disease.
Transcriptomics
Transcriptomics examines the complete set of RNA transcripts produced by the genome under specific circumstances or in a particular cell type. Technologies such as RNA sequencing (RNA-seq) allow researchers to measure gene expression levels and understand gene regulatory networks.
Proteomics
Proteomics is the large-scale study of proteins, including their structures, functions, and interactions. Mass spectrometry (MS) and protein microarrays are common techniques used to analyze the proteome, shedding light on the dynamic protein landscape.
Metabolomics
Metabolomics focuses on the comprehensive analysis of metabolites, the small molecules involved in metabolic processes. Techniques like nuclear magnetic resonance (NMR) spectroscopy and gas chromatography-mass spectrometry (GC-MS) provide insights into the metabolic state of cells and tissues.
Epigenomics
Epigenomics studies the chemical modifications on DNA and histone proteins that regulate gene expression without altering the underlying DNA sequence. Techniques such as bisulfite sequencing and chromatin immunoprecipitation sequencing (ChIP-seq) are used to explore epigenetic changes.
Integrative Multi-omics: Concept and Importance
Integrative multi-omics is the convergence of these diverse omics layers to create a unified, comprehensive view of biological systems. By combining data from genomics, transcriptomics, proteomics, metabolomics, and epigenomics, scientists can gain a deeper understanding of the intricate regulatory networks and pathways that govern life.
Advantages of Integrative Multi-omics
- Holistic View: Integrative multi-omics provides a more complete picture of biological systems by incorporating multiple layers of information.
- Data Integration: Combining different omics data sets can reveal new correlations and causal relationships that single-omics studies might miss.
- Personalized Medicine: Multi-omics approaches enable the identification of biomarkers for disease diagnosis, prognosis, and treatment, paving the way for personalized medicine.
- Drug Discovery: By understanding the multi-layered impact of drugs on biological systems, researchers can identify potential drug targets and develop more effective therapies.
Applications in Biomedical Research
Integrative multi-omics has numerous applications in biomedical research, significantly advancing our understanding of complex diseases and informing clinical practices.
Cancer Research
Cancer is a multifaceted disease characterized by genetic, epigenetic, transcriptomic, proteomic, and metabolic alterations. Integrative multi-omics helps unravel the complexity of cancer by identifying key drivers of tumorigenesis, discovering novel biomarkers for early detection, and uncovering therapeutic targets.
Neurodegenerative Diseases
Conditions such as Alzheimer’s and Parkinson’s disease involve complex interactions between genetic, epigenetic, and environmental factors. Multi-omics approaches can elucidate these interactions, providing insights into disease mechanisms and identifying potential therapeutic strategies.
Cardiovascular Diseases
Integrative multi-omics aids in understanding the molecular basis of cardiovascular diseases, identifying risk factors, and developing personalized treatment plans. For instance, multi-omics can reveal how genetic variants influence lipid metabolism and contribute to atherosclerosis.
Infectious Diseases
Multi-omics approaches are crucial for studying host-pathogen interactions, understanding immune responses, and developing effective vaccines and therapies. Integrative analysis of genomic, transcriptomic, proteomic, and metabolomic data can provide a comprehensive view of infection dynamics.
Challenges and Future Directions
While integrative multi-omics holds great promise, several challenges must be addressed to fully realize its potential. Tackling these challenges will not only advance the field but also ensure that the benefits of multi-omics research are broadly accessible and ethically sound.
Data Integration and Interpretation
Combining and interpreting data from different omics layers is a complex task. Advanced computational methods and machine learning algorithms are essential for integrating heterogeneous data sets and extracting meaningful insights. The sheer volume and diversity of data present significant challenges. Each omics layer—genomics, transcriptomics, proteomics, metabolomics, and epigenomics—produces vast amounts of data, often requiring different analytical approaches. Effective data integration must account for the variability and noise inherent in these datasets, ensuring that meaningful patterns are not obscured by irrelevant information.
Moreover, the development of robust bioinformatics tools and pipelines is critical. These tools must be capable of handling multi-dimensional data, performing high-throughput analyses, and providing user-friendly interfaces for researchers. Interdisciplinary collaboration between biologists, bioinformaticians, and computer scientists is vital to develop and refine these tools. Additionally, the incorporation of advanced visualization techniques can help researchers interpret complex data and communicate findings more effectively.
Standardization and Reproducibility
Standardizing protocols for sample collection, data generation, and analysis is crucial to ensure reproducibility and comparability of multi-omics studies across different laboratories and cohorts. Variability in experimental procedures, data processing methods, and analytical techniques can lead to inconsistent results, hampering the ability to draw reliable conclusions.
Efforts to develop standardized guidelines and best practices are underway, but widespread adoption is needed. These standards should cover all aspects of multi-omics research, from sample preparation and data acquisition to computational analysis and data sharing. Establishing centralized repositories for multi-omics data, along with detailed metadata, can facilitate data sharing and reproducibility. Additionally, promoting open access to multi-omics data and analytical tools will enable researchers worldwide to validate findings and build upon existing knowledge.
Cost and Accessibility
Multi-omics technologies can be expensive, limiting their accessibility to well-funded research institutions. Reducing costs and developing more affordable technologies will be key to democratizing access to multi-omics research. Advances in sequencing technologies, mass spectrometry, and other analytical techniques are gradually lowering costs, but further efforts are needed to make these technologies more affordable.
Funding agencies and research organizations can play a pivotal role by supporting initiatives aimed at reducing the cost of multi-omics research. Collaborative projects that pool resources and expertise can also help mitigate financial constraints. Additionally, fostering partnerships between academia, industry, and government can accelerate the development and dissemination of cost-effective multi-omics technologies. Ensuring that researchers from diverse backgrounds and regions have access to these tools is essential for fostering innovation and addressing global health challenges.
Ethical and Privacy Concerns
The integration of large-scale omics data raises ethical and privacy concerns. Ensuring the secure handling of sensitive genetic and health data and obtaining informed consent from participants are critical considerations. The potential for re-identification of individuals from anonymized omics data poses a significant risk to privacy. Implementing robust data encryption, secure storage solutions, and stringent access controls can help mitigate these risks.
Ethical considerations also extend to the responsible use of multi-omics data. Researchers must navigate complex issues related to data ownership, intellectual property, and the equitable sharing of benefits arising from multi-omics research. Establishing clear guidelines and ethical frameworks can help address these challenges, ensuring that the rights and interests of participants are protected.
Additionally, public engagement and education are vital to build trust and understanding of multi-omics research. Transparency in communicating the aims, benefits, and potential risks of multi-omics studies can foster public support and participation. Policymakers, ethicists, and researchers must work together to develop and implement policies that balance scientific advancement with ethical and privacy considerations.
Conclusion
Integrative multi-omics is revolutionizing our understanding of complex biological systems, breaking barriers that once seemed insurmountable. By merging data from various omics layers, researchers can gain a holistic view of biological processes, unravel disease mechanisms, and develop personalized treatments.
The comprehensive approach of integrative multi-omics has already demonstrated its immense potential in various fields. In cancer research, it has identified novel subtypes and therapeutic targets, offering new hope for precision oncology. In the realm of metabolic disorders, multi-omics studies have elucidated complex metabolic pathways, enabling the development of biomarkers for early diagnosis and targeted interventions. Neurodegenerative disease research has also benefited, with multi-omics uncovering new disease mechanisms and paving the way for innovative treatments.
Despite its promise, integrative multi-omics faces several challenges. Data integration and interpretation require sophisticated computational tools and expertise, while standardization of protocols is essential to ensure reproducibility and comparability. The cost of multi-omics technologies remains a barrier to widespread adoption, necessitating efforts to make these tools more affordable and accessible. Ethical and privacy concerns also need careful consideration, particularly when handling sensitive genetic and health data.
Looking ahead, the future of integrative multi-omics is bright. Technological advancements in sequencing, mass spectrometry, and data analysis are continually improving the accuracy and resolution of omics data. Machine learning and artificial intelligence are playing an increasingly important role in integrating and interpreting complex datasets, uncovering new insights that were previously beyond reach. Collaborative efforts across disciplines and institutions are fostering innovation and driving the field forward.
As we continue to push the boundaries of what is possible, integrative multi-omics will undoubtedly play a central role in advancing biomedical research, transforming healthcare, and improving patient outcomes. The journey is just beginning, and the potential for discovery is limitless. By embracing the power of integrative multi-omics, we are on the cusp of a new era in understanding and treating complex biological systems.
References
https://www.ncbi.nlm.nih.gov/pmc/articles/PMC2949280
https://www.ncbi.nlm.nih.gov/pmc/articles/PMC9289742
https://www.ncbi.nlm.nih.gov/pmc/articles/PMC2876223
https://www.ncbi.nlm.nih.gov/pmc/articles/PMC11120334
https://www.ncbi.nlm.nih.gov/pmc/articles/PMC9979795
https://www.ncbi.nlm.nih.gov/pmc/articles/PMC4850886
https://www.ncbi.nlm.nih.gov/pmc/articles/PMC5929475