Table of Contents
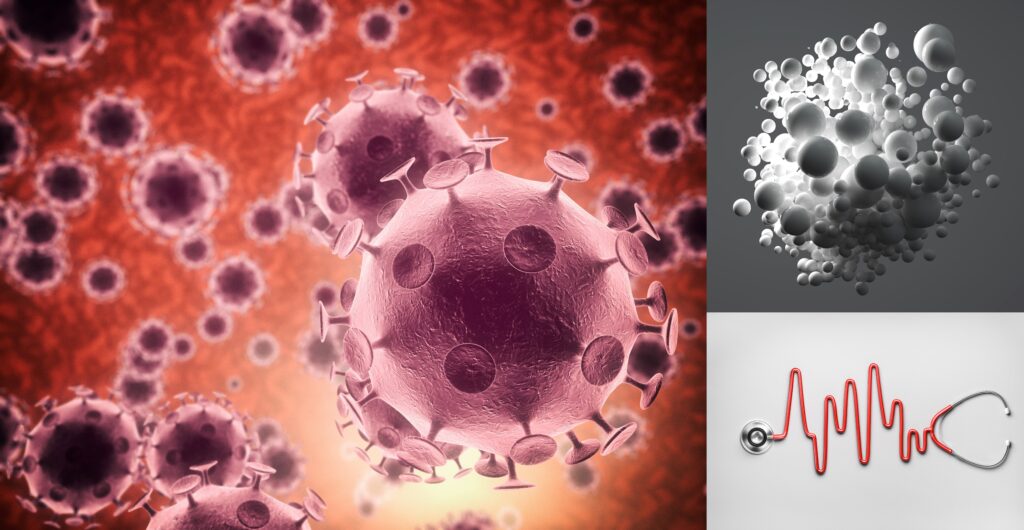
Introduction
Immunometabolism, a promising field at the intersection of immunology and metabolism, examines how metabolic processes influence immune cell function and vice versa. Understanding these intricate relationships is critical for developing novel therapeutic strategies for a variety of diseases, including cancer, autoimmune disorders, and infections. The immune system, our body’s defense mechanism, relies on a delicate balance of metabolic processes to function effectively. This balance is not only crucial for fighting off pathogens but also for preventing excessive inflammation and autoimmune reactions. This article will describe this immunometabolism, and uncover the potential for innovative treatments and improved health outcomes.
What is Immunometabolism?
Immunometabolism refers to the study of how metabolic processes within immune cells affect their function and how immune signals influence metabolic pathways. This dual regulation is crucial for maintaining homeostasis and effective immune responses. Metabolism, the sum of all biochemical reactions in the body, provides the necessary energy and building blocks for cellular activities. In immune cells, metabolic pathways are intricately linked to their activation, differentiation, and function. The interplay between metabolism and immunity is a dynamic process that adapts to various physiological and pathological conditions.
Metabolic Pathways in Immune Cells
Immune cells utilize different metabolic pathways to meet their energy demands and functional requirements. The two primary pathways are as below.
- Glycolysis: Rapid energy production through the conversion of glucose to pyruvate, predominantly used by activated immune cells. Glycolysis is advantageous for its speed, allowing immune cells to quickly generate ATP, the energy currency of the cell. However, this process is less efficient in terms of ATP yield compared to oxidative phosphorylation.
- Oxidative Phosphorylation (OXPHOS): A more efficient, albeit slower, energy production pathway through the mitochondrial electron transport chain, typically utilized by resting and long-lived immune cells. OXPHOS generates a higher amount of ATP per glucose molecule but requires a steady supply of oxygen, making it suitable for cells that do not need rapid energy production.
Key Immune Cells and Their Metabolic Preferences
- T Cells: Naive and memory T cells rely on OXPHOS to maintain their quiescent state and long-term survival. Upon activation, T cells switch to glycolysis to support rapid proliferation and effector functions. This metabolic reprogramming is essential for T cells to meet the high energy demands of clonal expansion and cytokine production.
- Macrophages: Inflammatory (M1) macrophages prefer glycolysis, which supports the production of pro-inflammatory cytokines and reactive oxygen species. In contrast, anti-inflammatory (M2) macrophages depend on OXPHOS and fatty acid oxidation to sustain their tissue repair and anti-inflammatory functions. This metabolic dichotomy allows macrophages to adapt to different microenvironments and functional states.
Metabolic Regulation of Immune Responses
Activation and Differentiation of Immune Cells
The metabolic state of an immune cell influences its activation and differentiation. For instance, T cell activation requires a metabolic shift from OXPHOS to glycolysis, supporting rapid cell growth and function. This shift is driven by signals from the T cell receptor (TCR) and costimulatory molecules, which activate pathways like PI3K/Akt and mTOR. These pathways promote glycolysis and biosynthetic processes necessary for T cell proliferation and effector functions.
Similarly, the differentiation of T cells into various subsets (e.g., Th1, Th2, Th17, Treg) is influenced by their metabolic state. Th1 and Th17 cells, which are involved in pro-inflammatory responses, rely heavily on glycolysis. In contrast, regulatory T cells (Tregs), which help maintain immune tolerance, depend more on OXPHOS and fatty acid oxidation.
Inflammatory Responses
Metabolic pathways also play a crucial role in shaping inflammatory responses. Pro-inflammatory cytokines are produced in glycolytic conditions, while anti-inflammatory cytokines are associated with OXPHOS and fatty acid oxidation. For example, the production of interleukin-1β (IL-1β), a key pro-inflammatory cytokine, is linked to glycolysis in macrophages. In contrast, the anti-inflammatory cytokine interleukin-10 (IL-10) is associated with OXPHOS.
The metabolic environment of immune cells can also affect their interaction with other cells and tissues. For instance, the accumulation of metabolic intermediates like succinate and itaconate in macrophages can modulate their inflammatory response and influence the outcome of infections and tissue repair.
Immunometabolic Disorders
Disruptions in immunometabolism can lead to various diseases. For example, metabolic dysfunction in immune cells is implicated in chronic inflammatory diseases, such as obesity and type 2 diabetes. In these conditions, immune cells often exhibit a pro-inflammatory metabolic profile, contributing to systemic inflammation and tissue damage. Similarly, metabolic abnormalities in immune cells are observed in autoimmune diseases, where dysregulated immune responses target the body’s own tissues.
Metabolic reprogramming of immune cells is also a feature of cancer. Tumor cells create a metabolically hostile environment by consuming large amounts of glucose and other nutrients, depriving immune cells of the resources needed for effective anti-tumor responses. Understanding the metabolic adaptations of immune cells in these diseases can provide insights into potential therapeutic strategies.
Therapeutic Implications of Immunometabolism
Cancer Immunotherapy
Targeting metabolic pathways in immune cells offers promising therapeutic strategies in cancer treatment. Enhancing glycolysis in T cells can boost their anti-tumor activity, while inhibiting tumor cell metabolism can reduce tumor growth. For example, metabolic checkpoint inhibitors, such as those targeting the enzyme PD-1, can reinvigorate exhausted T cells by modulating their metabolism.
Additionally, metabolic therapies can be combined with traditional treatments to improve outcomes. For instance, the use of ketogenic diets or drugs that alter nutrient availability can enhance the effectiveness of immune checkpoint inhibitors. Understanding the metabolic interplay between immune cells and tumor cells is crucial for developing comprehensive cancer therapies.
Autoimmune Diseases
In autoimmune diseases, modulating immune cell metabolism can help restore immune tolerance. For example, promoting OXPHOS in T cells can reduce their inflammatory potential and enhance the function of Tregs. Metabolic interventions, such as the use of metformin or other mitochondrial-targeted drugs, have shown promise in preclinical models of autoimmune diseases.
Moreover, understanding the metabolic triggers of autoimmunity can aid in the development of preventive strategies. For instance, dietary interventions or the use of probiotics to modulate gut microbiota can influence the metabolic profile of immune cells and reduce the risk of autoimmune reactions.
Infectious Diseases
Manipulating metabolic pathways in immune cells can also enhance responses to infections. For instance, enhancing glycolysis in macrophages can improve their ability to combat bacterial infections. Conversely, promoting OXPHOS in T cells can enhance their longevity and memory responses, improving vaccine efficacy.
Emerging therapies aim to harness the metabolic flexibility of immune cells to boost their antimicrobial functions. This approach includes the use of metabolic adjuvants in vaccines or the development of drugs that enhance the metabolic resilience of immune cells during infections.
Research advancements in Immunometabolism
Single-Cell Metabolomics
Advancements in single-cell metabolomics provide detailed insights into the metabolic states of individual immune cells, helping to understand their functional heterogeneity and responses. Single-cell techniques allow researchers to dissect the metabolic landscape of immune cells in different tissues and disease states, revealing new therapeutic targets.
For example, single-cell RNA sequencing combined with metabolic profiling can identify metabolic signatures associated with specific immune cell subsets. This approach can uncover the metabolic drivers of immune cell dysfunction in diseases like cancer and autoimmune disorders.
Metabolic Imaging
Novel imaging techniques allow for the visualization of metabolic processes in live cells and tissues, offering a real-time understanding of immunometabolic dynamics. Techniques such as fluorescence lifetime imaging microscopy (FLIM) and positron emission tomography (PET) provide non-invasive ways to study the metabolic activity of immune cells in vivo.
Metabolic imaging can also be used to monitor the effectiveness of metabolic therapies. For instance, changes in glucose uptake or mitochondrial function can be tracked to assess the impact of treatments on immune cell metabolism and function.
CRISPR and Gene Editing
CRISPR technology enables precise manipulation of metabolic genes in immune cells, facilitating the study of their roles in immune function and disease. By selectively knocking out or overexpressing specific genes, researchers can unravel the complex regulatory networks that link metabolism and immunity.
Gene editing tools also hold potential for developing novel therapies. For example, engineering T cells with enhanced metabolic properties can improve their persistence and efficacy in cancer immunotherapy. Similarly, editing metabolic genes in hematopoietic stem cells can create immune cells with tailored metabolic profiles for treating metabolic disorders and immune deficiencies.
Conclusion
Immunometabolism represents a critical nexus between metabolic processes and immune responses. Continued research in this field holds great potential for developing innovative treatments for a wide range of diseases, from cancer to autoimmune disorders and infections. Understanding and manipulating the metabolic pathways in immune cells can pave the way for precision medicine and improved therapeutic outcomes. The future of immunometabolism lies in integrating cutting-edge technologies and multidisciplinary approaches to unlock the full therapeutic potential of metabolic regulation in immune responses.
Frequently Asked Questions (FAQs)
1. What is immunometabolism?
Immunometabolism studies how metabolic processes within immune cells affect their function and vice versa.
2. How do metabolic pathways influence immune cell function?
Different metabolic pathways provide the necessary energy and resources for immune cell activation, differentiation, and function.
3. What is the role of glycolysis in immune cells?
Glycolysis provides rapid energy production, supporting the activation and function of immune cells like T cells and inflammatory macrophages.
4. How does immunometabolism affect inflammatory responses?
Metabolic states influence the production of pro-inflammatory and anti-inflammatory cytokines, shaping the overall immune response.
5. Can targeting metabolic pathways improve cancer treatment?
Yes, modulating metabolic pathways in immune cells can enhance their anti-tumor activity, offering potential therapeutic strategies in cancer immunotherapy.
6. What are the implications of immunometabolism in autoimmune diseases?
Modulating immune cell metabolism can help restore immune tolerance and reduce inflammation in autoimmune diseases.
7. How does immunometabolism relate to infectious diseases?
Manipulating immune cell metabolism can enhance their ability to combat infections, improving immune responses.
8. What are the latest research advancements in immunometabolism?
Advancements include single-cell metabolomics, metabolic imaging, and CRISPR gene editing, offering new insights and tools for studying immunometabolism.
References
https://www.ncbi.nlm.nih.gov/pmc/articles/PMC4710204
https://www.nature.com/articles/s41423-022-00840-x
https://www.ncbi.nlm.nih.gov/pmc/articles/PMC5800786
https://www.nature.com/articles/s41418-022-01022-y
https://www.mdpi.com/1422-0067/24/5/4954
YouTube Linkhttps://youtube.com/shorts/nqDQu6b_pOk?feature=share